Effect of Histamine on the Retina
ABSTRACT
The retina has long been considered a unidirectional circuit sending visual input to the brain. A recent discovery alters this dogma: In adult mice, histaminergic neurons from the hypothalamic region of the brain project to the retina and modulate its neural activity. This discovery has implications for the formation of the visual system because retinal activity is known to drive its development. However, it is unknown if histamine innervates and modulates the retina during its development. Here, a multi-electrode array (MEA), a device that records the neural activity of hundreds of neurons at once, was used to determine when and how histamine begins to modulate the developing mouse retina. In the first postnatal week, histamine has no effect on neural activity in the retina. At the start of the second postnatal week, histamine does show a noticeable effect on the neural activity of the retina. These results indicate that histamine is involved in the development of the visual circuit and given that histamine release is perturbed by chronic anxiety and sleep deprivation, our results have implications on the further understanding of healthy and unhealthy development of the visual system.
INTRODUCTION.
Vision is one of the five key senses. The retina, located at the back of the eye, is the first location where the nervous system transduces photons of light into a neural signal. The main function of the retina is to detect general aspects of the visual scene (e.g.: amount of light, color, motion) and transfer this information to the rest of the brain, like the visual thalamus, colliculus, and cortex [1]. Previously, this flow of information was believed to be one-directional, but a recently discovered projection from the brain to the eye upends this belief [2].
As recently reported, histaminergic neurons which originate in the tuberomammillary nucleus (TMN) of the hypothalamus in the brain have been seen to innervate the retina in adult mice [2]. Projections of histamine to the retina were previously observed in rat and monkey retinal ganglion cells (RGC), which are the neurons in the retina that project to the brain [3]. However, this was the first projection to be found in the mouse after Greferath et al., 2014 had found no evidence of histamine in the mouse retina [4]. In the nervous system, histamine acts as a neurotransmitter and is only synthesized in the TMN [5]. Although the retina cannot produce histamine, retinal histamine levels are comparable to histamine levels in the rest of the nervous system and in the recent discovery, it was also seen that histaminergic neurons modulate different RGC subtypes [2,6]. Therefore, histamine seems to have an important role in the retina, however this role has not been tested during development.
The development of the visual system depends on neural activity, some of it produced spontaneously in the form of retinal waves. Retinal waves are correlated electrical activity propagating throughout the retina during development beginning embryonically in mice and lasting until eye opening (14 days after birth) and these waves have different stages mediated by various retinal circuits [7]. Retinal waves have been associated with the development of many pathways in the visual circuit. For example, it has been shown that retinal wave properties such as the asynchrony of waves instruct the development of eye-specific segregation [8]. Other aspects of visual development, like how the visual brain is organized and our ability to detect motions, also seem to be instructed by different spatial-temporal properties of waves during development [9].
Therefore, given that histamine modulates the retina and that retinal activity during development is critical for the proper wiring of the visual nervous system, histamine modulation of the retina is likely important for development. Therefore, in this initial study of histamine, it was tested when histamine begins to modulate the mouse retina during development as well as how histamine modulates the retina in order to get a basic understanding of the mechanisms involved in visual circuit development.
MATERIALS AND METHODS.
Animals.
Twelve C57B6 mice were utilized for the study. Mice were anesthetized using isoflurane and then were euthanized by decapitation. For retina preparation, the methodology described previously was followed [10]. In brief, eyes were immediately enucleated, and retinas were dissected out of the eye under a stereo microscope. Then relief cuts were administered on the retina to make the retina flat and suitable for the Multi-Electrode Array. The retina was kept in oxygenated Artificial Cerebrospinal Fluid (ACSF) at 33°C to imitate the conditions found inside of the mouse.
Multi-Electrode Array Setup.
A Maxwell Biosystems MaxOne multi-electrode array (MEA) was used to record the retina’s activity, including the electrical activity of retinal waves. The MEA records neural activity by recording electrically active cells through the electrodes present in the chip. Neurons exhibit action potentials, which is when the membrane potential of a cell rapidly changes, and the MEA picks up on these action potentials and records them. Oxygenated ACSF at 33°C was used in the MEA chip to replicate in vivo conditions for the retina. To ensure a good cycling of oxygenated ACSF into the MEA chip, the flow of ACSF was kept at 2mL/min.
Histamine Application.
Each of the retinas tested was put on the MEA for 10 minutes to record the baseline neural activity. After 10 minutes, 20 µM of histamine was added to the inflow and once histamine entered the chip, the recording for the neural activity after histamine was started and lasted for 10 minutes [2]. In total, there were 6 different ages of mice tested (one at 2 days old which is P2, one at 4 days old which is P4, three at 5 days old which is P5, three at 7 days old which is P7, three at 10 days old which is P10, and one at 13 days old which is P13) were used in this study to see histamine’s effect over time on the retinal activity.
Data Analysis.
MATLAB R2023a was used to analyze the dataset collected by the MEA. For all analysis, a first pass filter was used to remove quiet channels, which are channels that were not active during both the baseline and histamine recording, likely because the electrodes represented by these channels never made contact with a neuron. To classify these channels, a threshold was used. The threshold was the median of the control dataset, and that number was then applied to the histamine dataset of the same age to eliminate the quiet channels. These quiet channels were classified as channels that did not meet the same threshold in both the control/baseline and histamine datasets when it came to the number of times a neuron fired in the 10 minutes.
To assess whether histamine affected activity in the whole retina, the discrete action potential data was first converted into a continuous firing rate (spikes/second). Then, the firing rates of retinal neurons before and after application of histamine were plotted. MEA data was also used to assess whether histamine affected the amplitude of action potentials. The amplitude of all action potentials for each individual neuron in both the pre and post condition were averaged out. Then each neuron’s average amplitude was plotted on a scatter plot to compare the distributions for both conditions to determine if histamine affected the amplitude of action potentials. The firing rate for each individual neuron was also averaged out for both conditions to assess how histamine affected individual retinal ganglion cells. Then corresponding neurons from both datasets were taken and the average firing rate from the histamine condition was divided by the average firing rate from the pre-histamine condition. If the result was greater than 1, then histamine increases retinal firing rate and if the result was less than 1, then histamine decreases in firing rate. After compiling those values, the percentage of values that were greater than 1 were calculated, which gave the percentage of RGC’s that increased their firing rate.
RESULTS.
The overall activity across many neurons of the retina was first analyzed to gain a general understanding of when histamine begins to modulate the retina during development. In the first postnatal week, before postnatal day 7 (P7), applications of histamine do not noticeably modulate retinal activity (Figure 1A). However, in the second postnatal week, after P7, applications of histamine did noticeably modulate retinal activity (Figure 1B), and the effect only increased with mouse age. Specifically, histamine increased retinal activity by 1.91-fold at P13 compared to a 0.77 fold decrease at P2. Overall, histamine does not impact the number of waves occurring in the retina, characterized by the larger spikes in Figure 1B. Instead, histamine elevates the overall baseline neural activity, the spontaneous activity in the retina that does not result in retinal waves, at P10 (Figure 1B).
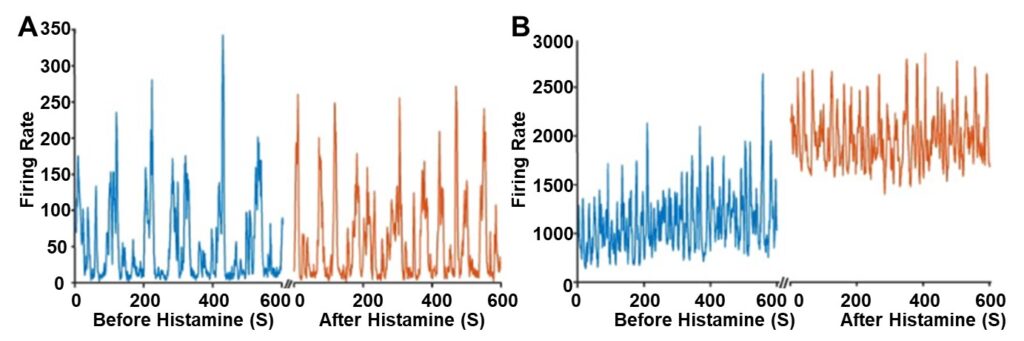
Next, histamine’s effect on individual neurons was analyzed to better understand how histamine is modulating retinal activity during development (Figure 2). In the first postnatal week, some RGCs exhibited a response to histamine but overall, the distribution of their firing was centered at no effect (Figure 2, Figure 3). However, in the second postnatal week, more than 50 percent of RGCs in the retina responded to histamine application with an increased firing rate (Figure 2, Figure 3). Specifically, at P5, on average, only 48.85% of neurons experienced an increase in their firing rate after histamine application but at P10, on average, 75.53% of neurons experienced an increase in their firing rate after histamine application.
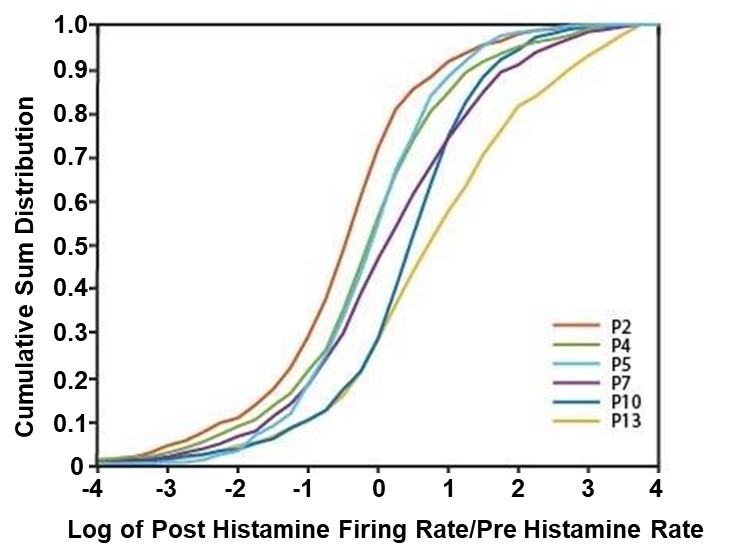
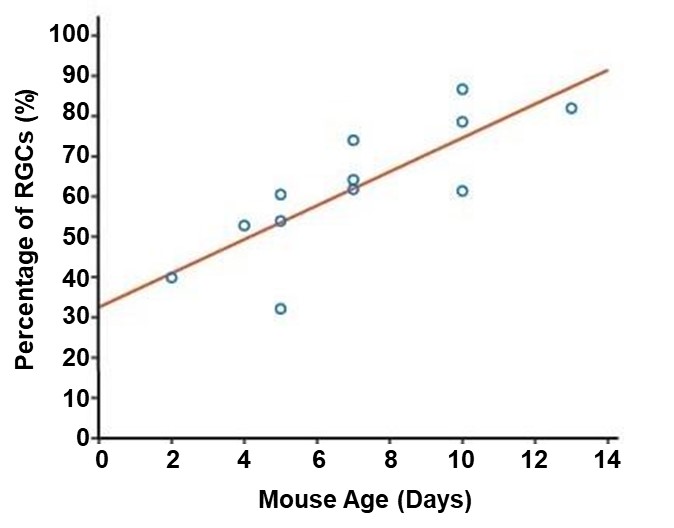
DISCUSSION.
In this study, we established when histamine begins to modulate the retina and how it affects neural activity in the developing retina. We report that histamine innervation increases the spontaneous activity in the retina starting from P7.
Histamine Receptors.
There are 4 different histamine receptors present in mammals: H1R, H2R, H3R, and H4R [11]. These histamine receptors all have different structures and functions. This study has shown that there is histamine innervation in the developing retina. Therefore, finding which histamine receptor is present in the developing retina will be important to find the exact role of the histamine innervation in the retina and is an avenue for future exploration. Receptors H1R and H2R are associated with mostly excitatory actions while H3R is associated with mostly inhibitory actions [12]. To test this, drugs such as dimetindene maleate can be used to block H1R and administer histamine to the retina and observe the effect on the retina [2]. This could be done on other receptors with other receptor blockers to observe the effect of histamine on the retina to determine the relative contributions of each histamine receptor. Determining which histamine receptors are involved in the development of the visual system may reveal novel therapeutic targets.
Eye Specific Segregation.
Eye specific segregation is the segregation of inputs in the primary visual cortex from the two different eyes. Neurons become specialized to process information from a specific eye in this process. Eye specific segregation starts early during development and is mediated by retinal waves. It has been shown that asynchronous activity between the eyes and precise temporal pattern of retinal waves regulate eye-specific segregation [8]. When histamine was applied, an increase was seen in the general activity of the retina so it is plausible that histamine affected the synchrony and temporal pattern of waves, and therefore might have an effect on the eye-specific segregation [8]. To test further, histamine’s effect on the synchrony and temporal patterns of retinal waves should be examined to determine histamine’s impact on eye-specific segregation.
Retinotopic Mapping.
Retinotopic mapping is the organization of neurons in the primary visual cortex according to the layout of neurons in the retina. This mapping is essential for the brain to make sense of visual input and this mapping also starts during development and is mediated by retinal waves [9]. It has been shown that the specific size of retinal wave propagation is important for the development of retinotopic mapping [9]. When histamine was applied, a general increase in activity was seen but the waves themselves were not looked at especially if they were larger or not. To test further, histamine’s effect should be examined on the size of retinal waves to investigate histamine application on correct mapping of the visual system.
Direction Selectivity.
Some neurons have a preferred direction of motion and exhibit a stronger response when a stimulus comes in that direction [13]. These direction-selective neurons are critical for the brain’s ability to detect motion and the direction of motion. Again, the development for this process is mediated by retinal waves. Specifically, it has been shown that the directionality of waves or the propagation bias of waves has an effect on the emergence of direction selective neurons [13]. With histamine’s noticeable effect on retinal waves in the second postnatal week, it is plausible that the effect on retinal waves includes affecting the direction that they propagate in. Therefore, it is important to test whether histamine has an effect on propagation bias of waves in order to determine its effect on direction selectivity.
Effect on Sleep/Wake Cycle.
Histaminergic neurons in the nervous system have been seen to be associated with the sleep/wake cycle [14]. Histaminergic neurons are most active during arousal/wake while they are least active during sleep [15]. Perhaps coincidentally, developing mammals spend a disproportionate amount of time asleep and several theories argue that sleep plays important roles during development [16]. Given that our results show that histamine alters retinal activity, perhaps a role of sleep is to maintain low levels of histamine in the retina so that retinal waves can be the main type of activity. This implies that sleep deprivation could potentially be bad for the development of the visual system, though this idea still needs to be tested.
CONCLUSION.
In conclusion, histamine begins to modulate the neural activity in the developing retina starting from P7. We also report that histamine innervation increases the spontaneous activity in the retina starting from P7.
ACKNOWLEDGMENTS.
I would like to thank Dr. Alexandre Tiriac for letting me be part of this team. I would also like to thank Dr. Dominic Vita for his guidance. I would also like to thank the SSMV for the opportunity to pursue research and Dr. Menton Deweese for being my advisor in this process.
REFERENCES
- Kerschensteiner D, Feature Detection by Retinal Ganglion Cells. Annual Review of Vision Science. 2022;8(1):135-169.
- Warwick RA, et al. Top-down modulation of the retinal code via histaminergic neurons of the hypothalamus. Cold Spring Harbor Laboratory Press; 2022.doi:10.1101/2022.04.26.489509.
- Gastinger MJ, et al. The Effects of Histamine on Rat and Monkey Retinal Ganglion Cells. Visual Neuroscience. 2004;21(6):935-943.
- Greferath U, et al. The Role of Histamine in the Retina: Studies on the Hdc Knockout Mouse. PLoS ONE. 2014;9(12):e116025-e116025.
- Airaksinen MS, Panula P. The histaminergic system in the guinea pig central nervous system: An immunocytochemical mapping study using an antiserum against histamine. Journal of comparative neurology (1911). 1988;273(2):163-186.
- Arbonés L, et al. Presence and distribution of histaminergic components in rat and bovine retina. Neurochemistry international. 1988;13(1):97-104.
- Thompson A, et al. Activity-dependent development of visual receptive fields. Current opinion in neurobiology. 2017;42:136-143.
- Zhang J, et al. Visual map development depends on the temporal pattern of binocular activity in mice. Nature neuroscience. 2012;15(2):298-307.
- Xu H ping, et al. An Instructive Role for Patterned Spontaneous Retinal Activity in Mouse Visual Map Development. Neuron. 2011;70(6):1115-1127.
- Tiriac A, et al. The influence of spontaneous and visual activity on the development of direction selectivity maps in mouse retina. Cell reports. 2022;38(2):110225-110225.
- Gastinger MJ, et al. Histamine receptors in mammalian retinas. Journal of comparative neurology. 2006;495(6):658-667.
- Haas H, Panula P. The role of histamine and the tuberomamillary nucleus in the nervous system. Nature reviews Neuroscience. 2003;4(2):121-130.
- Ge X, et al. Retinal waves prime visual motion detection by simulating future optic flow. Science. 2021;373(6553).
- Scammell TE, et al. Histamine: neural circuits and new medications. Sleep. 2019;42(1).
- Takahashi K, et al. Neuronal Activity of Histaminergic Tuberomammillary Neurons During Wake-Sleep States in the Mouse. The Journal of neuroscience. 2006;26(40):10292-10298.
- Blumberg MS, et al. Perspective Sleep, plasticity, and sensory neurodevelopment. Neuron. 2022;110(20):3230-3242.
Posted by buchanle on Tuesday, April 30, 2024 in May 2024.