The Development of synNotch-Engineered Pluripotent Stem Cells for Synthetic Morphogenesis
ABSTRACT
Pluripotent stem cells can expand and differentiate into various cell fates, making them a valuable tool for tissue engineering. WNT is a morphogen essential for early embryonic development by differentiating and patterning the germ layers. Previous studies have researched WNT signaling and its effect on development using bulk media supplementation of recombinant factors and small molecules. In nature, these events are cell-mediated, so the synthetic Notch platform (synNotch) was utilized, allowing cells to respond to immobilized ligands on an engineered substratum, thereby achieving desired gene expression, investigating the role of cell-secreted morphogens. Additionally, two WNT transgenes, mWNT3a and a WNT surrogate, were examined to see how signaling range may influence differentiation in H9 human embryonic stem cells (hESCs). Two hESC lines were engineered to express a GFP-responsive synNotch receptor so that in the presence of immobilized GFP, synNotch activation would result in the expression of one of the WNT transgenes and the fluorescent reporter mCherry. Overall, ligand-inducible differentiation of synNotch-hESCs was achieved, showing that the cells secreted sufficient levels of WNT for differentiation. Additionally, the WNT surrogate synNotch-hESCs displayed more dispersed brachyury expression. These findings may propose an alternative method for dosing cells with morphogens that more closely mimic nature.
INTRODUCTION.
Pluripotent stem cells (PSCs) have two main functions: the ability to self-renew and, through morphogenesis, differentiate into numerous different cell types [1]. Due to these unique abilities, stem cells can be used to fight diseases and repair damaged areas in the body, making them a great contender for tissue engineering. An early form of stem cell differentiation occurs during gastrulation. Gastrulation is an early developmental process that gives rise to and patterns the three germ layers the endoderm, mesoderm, and ectoderm from the pluripotent epiblast [2]. This process is orchestrated by three morphogens: BMP, WNT, and NODAL [3]. The germ layers prime cells to become more specialized cells during embryonic development. For example, the ectoderm primes stem cells towards neuronal fates [2].
During early embryonic development, the morphogen WNT helps initiate the differentiation and patterning of the germ layers as well as giving rise to the primitive streak, establishing the body axis for the rest of development [4]. WNT3a is a member of the WNT family that activates the WNT/ β-catenin signaling pathway and is implicated in embryonic and neural development [5]. WNT3a occurs naturally and has a relatively short signaling range [5]. To extend the signaling range of WNT3a, a WNT surrogate was developed using protein engineering [6], resulting in an extended signaling range [7]. Thus, it can be used to investigate how the signaling range of WNT may influence development.
WNT signaling dynamics are conventionally challenging to control in vitro [8]. Synthetic biology tools, including genetic engineering, can help create better biomimetic models. synNotch is a synthetic version of the native Notch-Delta contact-dependent signaling channel [9] (Figure 1A). synNotch is a modular platform containing a user-defined extracellular and intracellular domain with a conserved transmembrane Notch region [9]. The modular nature of synNotch enables cells to respond to specific immobilized ligands to obtain desired transgene expression. In the presence of an immobilized ligand, the conserved Notch region is cleaved, releasing a transcription factor that translocates to the nucleus, resulting in downstream gene expression (Figure 1B).
Due to technical challenges associated with studying gastrulation in vivo, 2D in vitro models that leverage PSC technology have been established and used to investigate morphogen signaling pathways [13], [14]. These studies have greatly contributed to understanding early embryonic development; however, they often rely on bulk media supplementation of morphogens which do not mimic the cell-mediated events occurring in nature [13]. It was hypothesized that by engineering stem cells with synNotch, WNT signaling dynamics during early embryonic development could be investigated in a more biomimetic manner in vitro. Therefore, H9 hESCs were engineered with synNotch to respond to the bioinert cue, GFP, to produce the morphogen WNT themselves. This enabled the investigation of how cell-secreted morphogens impact gastrulation differentiation and the role of morphogen signaling range by comparing mWNT3a and WNT surrogate. With the information learned from this experiment, this study has contributed to the field by highlighting the signaling dynamics of WNT and its effect on early gastrulation differentiation. Furthermore, these results and the associated platform have applications in tissue engineering to help develop more reproducible biomimetic tissues.
MATERIALS AND METHODS.
PSC maintenance.
H9 hESCs were kept in mTeSR Plus (Stem Cell Technologies, #: 100-0276) medium which was replaced every other day in a Geltrex-coated (Fisher Scientific, #: 14190144) 6-well plate. When cells were 70-80% confluent, they were rinsed with PBS and passaged with ReLeSR (Stem Cell Technologies, #: 100-0483). After incubation, mTeSR Plus was added to the well to lift cells which were plated in a new Geltrex-coated well.
For experiments, cells were passaged with Accutase (Thermo Fisher, #: A1110501) to obtain a single-cell suspension. The Accutase passaging consisted of four steps: dissociation, centrifugation, cell counting, and plating cells. Cells were rinsed with PBS before adding Accutase and incubating the cells at 37°C for 5 minutes. The reaction was then quenched with an equal volume of mTeSR Plus before being spun down at 300 x gravity for 5 minutes. The supernatant was removed, and cells were resuspended in mTeSR Plus supplemented with 10 µM of the rho kinase inhibitor Y-27632 (ROCK inhibitor) (Tocris, #:1254) and counted. Cells were then plated for experiments at 1.25 x 105 cells/cm2.
Establishing synNotch hESC lines.
To engineer the three synNotch-hESC lines, Snapgene software was utilized to design the plasmids. All plasmids contained a synNotch-engineered non-fluorescent GFP-responsive receptor that activated in response to the immobilized GFP ligand. The synNotch receptor also contained a myc tag to detect synNotch receptor expression in engineered cells. The plasmids contained either mWNT3a or the WNT surrogate in addition to an mCherry fluorescent reporter. The plasmid also contained a puromycin resistance gene to enable selection of engineered hESCs.
The plasmids were assembled via isothermal assembly using NEBuilder HiFi DNA assembly mix with an insert-to-backbone molar ratio of 2:1. The backbone fragment contained the synNotch receptor and the insert contained the transgenes. E. coli was transformed with the assembled plasmids and allowed to grow on an agar plate overnight. A colony PCR was performed to screen the bacteria and verify the presence of the plasmid of interest. Positive colonies were grown up overnight in LB broth before purifying the DNA with the Qiagen miniprep kit.
The Sleeping Beauty Transposon System was utilized to non-virally transfect the hESCs with the purified plasmids using the TransIT®-LT1 transfection reagent [15]. The purified plasmid, which contained the synNotch platform, was mixed with a transposase plasmid, containing the enzyme SB100x at a 1:1 ratio. The transposase bound to the repeated sections on either side of the gene of interest, cutting and releasing the transposon that contained the synNotch platform. The transposon was then inserted into the DNA of the cell. Engineered cells were then selected with puromycin since the plasmid contained puromycin resistance.
Engineered substratum.
Since synNotch requires an immobilized ligand to activate and stem cells require a basement membrane coating for culture in monolayer, an engineered substratum [11] was utilized. To prepare the substratum, 10 µg/mL of streptavidin was added to a non-tissue culture treated plate and incubated overnight. The peptide mixture used in the substratum consisted of two adhesion peptides, GBP (Genscript, custom peptide synthesis) [16] and cRGD (Fisher Scientific, #: 50-168-6291) [17], and a ligand-capturing polyethylene glycol (PEG) GFP-TRAP motif. [11] All three peptides contained biotinylated PEG linkers so that they would bind with a high affinity to the streptavidin coating. The peptides were diluted to a final concentration of 5 µM GBP, 2.15 µM cRGD, and 0.4 µM PEG GFP-TRAP. After removing the streptavidin (Thermo Scientific, #: 21125B) from the plate, the diluted peptide mixture was added and incubated at 37°C for an hour. The peptide mixture was then removed, and the cells were plated in the coated wells.
Flow cytometry.
To quantify receptor expression, flow cytometry was performed. Cells were dissociated with Accutase and resuspended in blocking buffer consisting of 5% FBS in PBS. The cells were then incubated on ice for 15 minutes. Cells were then stained with an Alexa Fluor 647 fluorophore conjugated to an anti-c-myc-tag (Cell Signaling Technology, #:2233S) at a 1:50 dilution in the blocking buffer on ice for 1 hour in the dark. An unstained control cell suspension was also prepared and left on ice. The cells were then rinsed twice with PBS before the samples were run through the Cellstream flow cytometer. Data was analyzed with Flowjo software. Cells were gated for the cell population, single cells, and then c-myc epitope tag (synNotch) positive.
Microscopy.
A Leica DMi8 microscope was used for phase contrast and fluorescence microscopy. All images were taken at either 10x or 20x.
Gastrulation differentiation.
For gastrulation differentiation, cells were kept in HUESM mouse embryonic fibroblast (MEF) conditioned medium (HUESM-CM) [13]. The medium was supplemented with 20 ng/mL of bFGF and 10 µM ROCK inhibitor. A subset of the wells was treated with 5 nM non-fluorescent soluble GFP (a mutant form of GFP that does not fluoresce, enabling use of the 488 nm channel for detecting alternative molecules). The medium was replaced 24 hours after plating, and immunofluorescent staining was performed after 48 hours.
Immunofluorescence.
To assess stem cell differentiation, immunofluorescent staining was performed. Cells were fixed with 4% paraformaldehyde. After 15 minutes, the cells were rinsed thoroughly with PBS before adding blocking and permeabilization buffer (5% FBS and 0.3% TritonX in PBS). Cells were left at room temperature to incubate for 30 minutes. Cells were stained with a rabbit anti-human VEGFR2 (Cell Signaling Technology, #: 9698S) (1:500 dilution) primary antibody and a goat anti-human brachyury (R&D Systems, #: AF2085) (1:200 dilution) primary antibody and incubated overnight at 4°C. The primary antibody solution was then removed, and the wells were rinsed with PBS twice. The wells were then stained with the secondary antibodies, donkey anti-rabbit Alexa Fluor 488 (Thermo Scientific, #: A21206) (1:500 dilution) and donkey anti-goat Alexa Fluor 647 (Thermo Scientific, #: A21447) (1:500 dilution). Cells were also counter-stained with the nuclear stain DAPI (Thermo Scientific, #: 62247) (1:1000 dilution). The plate was protected from light and allowed to incubate for 1 hour at room temperature. The secondary antibodies were then removed and rinsed twice with PBS. The permeabilization buffer was added to the cells for the imaging session.
RESULTS.
synNotch receptor expression and activation.
Flow cytometry was performed on the transfected hESCs to quantitatively gauge synNotch receptor expression of the engineered cells. Transfected hESCs were first treated with puromycin to enrich the synNotch-engineered hESC population. A subset of the cells was stained for the c-myc epitope tag present on the synNotch receptor to verify receptor expression and the remaining cells were used as an unstained control. Cells were gated into a single-cell population to remove debris and cell clumps. The single-cell population of stained cells showed a rightward shift in synNotch receptor expression compared to the unstained control, indicating the presence of the synNotch receptor on the engineered cells. Of the stained mWNT3a-engineered cells, 99.4% were found to be positive for expression of the synNotch receptor (Figure 2A). Of the stained WNT surrogate engineered cells, 97.3% were positive for synNotch receptor expression (Figure 2B). This suggested that the synNotch-engineered population of the hESCs made up the vast majority of the cell population for both the mWNT3a and WNT surrogate synNotch-engineered hESC cell lines.
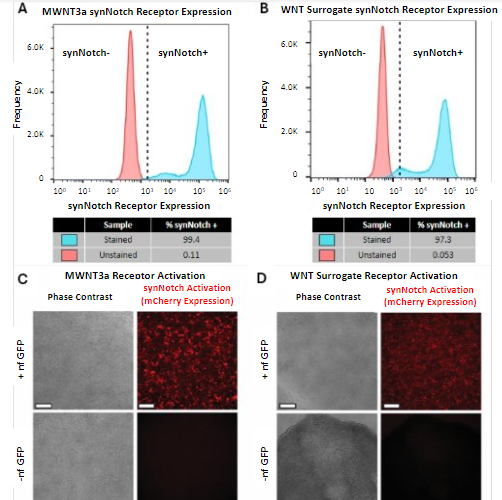
With the confirmation that most of the hESCs had been successfully transfected with the synNotch receptor platform, the synNotch-engineered hESCs were plated on a tri-peptide engineered substratum [11] composed of two adhesion peptides and one ligand capturing motif to immobilize GFP. The synNotch-engineered hESCs were then put through a modified 48-hour gastrulation differentiation protocol [13] to see if this tri-peptide surface would support the synNotch-engineered hESCs and activate the morphogenetic program. The cells were either maintained in HUESM-CM medium containing GFP or medium without. After 48 hours, the hESCs were imaged to assess stem cell adherence and synNotch activation via mCherry fluorescence. Both the mWNT3a (Figure 2C) and the WNT surrogate (Figure 2D) receptor cell lines showed adherence to the engineered substratum in a monolayer, though the WNT surrogate cells that were not treated with GFP did show signs of contraction. Only the wells supplemented with GFP showed robust mCherry expression, indicating that synNotch receptor activation for both synNotch-engineered hESCs occurred in an activating ligand-dependent manner.
Differentiation.
To gauge whether hESCs engineered with synNotch could drive sufficient production of WNT to initiate early gastrulation differentiation, the mWNT3a and WNT surrogate synNotch-engineered hESCs were stained for differentiation makers via immunofluorescent staining. After the 48-hour gastrulation differentiation protocol, the synNotch-engineered hESCs were fixed and stained for three markers: DAPI, a nuclear stain, VEGFR2, a mesendodermal marker, and brachyury, a primitive streak and mesendodermal marker. All wells showed ample DAPI staining, indicating the presence of cells in a monolayer. The wells kept in HUESM medium containing GFP showed more VEGFR2 and brachyury expression compared to wells maintained in HUESM medium without GFP. This suggests that, in response to the activating ligand, the synNotch-engineered cells produced sufficient amounts of WNT to initiate early gastrulation differentiation. The non-treated GFP negative group of the WNT surrogate did have more background fluorescence compared to the other images, likely due to the partial contraction of the cells around the edge. Furthermore, when looking at the entire well, the brachyury expression in the mWNT3a synNotch-engineered hESCs (Figure 3A) had more clusters of positive signaling, whereas the brachyury in the WNT surrogate synNotch-engineered hESCs (Figure 3B) had more dispersed expression, suggesting that the WNT surrogate may have an extended signaling range. These results imply that synNotch can drive the production of morphogens and initiate stem cell differentiation in an activating ligand-dependent manner.

DISCUSSION.
Here a platform was presented that enabled further research into morphogenesis using synthetic biology tools. H9 hESCs were successfully transfected with the synNotch receptor in both the mWNT3a and WNT surrogate cell lines. The transfected hESCs showed a high percentage of synNotch-positive cells from the total cell population, indicating that the cells expressed the synNotch receptor and had the potential to produce morphogens in subsequent experiments. The synNotch-engineered hESCs successfully adhered to an engineered substratum [11] in monolayer and achieved ligand-inducible synNotch receptor activation as seen through high levels of mCherry in the medium containing GFP. This helped verify that the receptors expressed by the engineered cells were working in the presence of the activating ligand. After confirming most of the engineered cells expressed the receptor and showed GFP-dependent activation, it was found that synNotch-engineered hESCs could drive the production of the morphogen WNT. After 48 hours, activated synNotch-engineered hESCs maintained in medium supplemented with GFP-expressed mesendodermal and primitive streak markers, indicating a transition toward an early gastrulation state. This supported the hypothesis that stem cells could be
engineered with a synNotch receptor to drive the expression of WNT and initiate differentiation to investigate WNT signaling dynamics in vitro with cell-secreted morphogens. Additionally, a comparison of the mWNT3a and WNT surrogate transgenes showed that brachyury expression was more dispersed in the WNT surrogate well. This suggested that the WNT surrogate may have an extended signaling range and that the signaling range of WNT influences stem cell differentiation.
Morphogenesis in nature is very complex and is dependent on morphogen concentration and the duration of morphogen signals [18]. It involves intricate signaling events that tightly control cell patterning and differentiation [14]. It can be artificially studied within the laboratory with the usage of engineering techniques, leading to the rise of synthetic morphogenesis [16]. Previous studies investigating morphogenesis have greatly contributed to the field; however, they lack spatial control with the bulk supplementation of soluble factors to replicate the effects of morphogens [13], [14]. By combining synthetic biology tools with what has been learned from these previous experiments, cell-mediated signaling events occurring during natural development is mimicked more closely. By engineering hESCs with the synNotch receptor and combining them with the engineered substratum, a platform capable of investigating morphogenesis synthetically is exhibited. This platform can initiate artificial morphogen signals via GFP-dependent expression of both mWNT3a and WNT surrogate. Furthermore, its potential to investigate differences in signaling dynamics is shown here with variable brachyury expression in WNT transgenes with different signaling ranges.
A major limitation in tissue engineering is the reproducibility of tissues. The synthetic platform described here utilized cell-mediated signaling and has potential for improved spatial control via patterning of the engineered substratum. Thus, this platform offers a chance to improve the reproducibility of differentiated cells through cell-mediated synthetic signaling and spatially controlled activation. Here proof-of-concept of a synthetic biology approach to investigate morphogenesis using material-mediated signaling in synNotch-engineered hESCs is shown [11]. Furthermore, WNT signaling dynamics during early embryonic development were investigated. In the future, this platform will be extended to micropatterned cell culture to investigate germ layer patterning in a spatially constrained manner. Additionally, the platform will be used to investigate morphogenetic events for different, longer-term differentiation protocols. Overall, this synthetic platform was used to show that H9 hESCs could be engineered with the synNotch receptor, synNotch-engineered hESCs could activate in a ligand-dependent manner on the substratum, synNotch-engineered hESCs could achieve differentiation of cells via synNotch-mediated morphogenetic programs, and that there may be differences in two WNT transgenes.
ACKNOWLEDGMENTS.
I would like to thank the Brunger Lab at Vanderbilt University and the School for Science and Math at Vanderbilt for the opportunity to conduct this research and support given throughout this process. This project was funded by NSF RECODE CBET-2033800 & NSF GRFP Grant No. 1937963.
REFERENCES.
[1] W. Zakrzewski, M. Dobrzyński, M. Szymonowicz, Z. Rybak, Stem cells: past, present, and future. Stem Cell Res. Ther. 10,1 68. (2019).
[2] E. Camacho-Aguilar, A. Warmflash, Insights into mammalian morphogen dynamics from embryonic stem cell systems. Curr. Top. Dev. Biol., 137, 273-305 (2020).
[3] J. Muhr, T. Arbor, K. Ackerman, Embryology, Gastrulation. StatPearls, StatPearls Publishing (2023).
[4] F. Tepekoy, G. Akkoyunlu, R. Demir, The role of Wnt signaling members in the uterus and embryo during pre-implantation and implantation. J. Assist. Reprod. Genet. 32(3), 337-346 (2015).
[5] S. He, et.al., WNT3A: Functions and implications in cancer. Chin. J. Cancer. 34(12), 554-562 (2015).
[6] C. Y. Janda, et.al., Surrogate Wnt agonists that phenocopy canonical Wnt and β-catenin signalling. Nature. 545(7653), 234-237 (2017).
[7] Y. Miao, et.al., Next-generation surrogate WNTS support organoid growth and Deconvolute frizzled pleiotropy in vivo. Cell Stem Cell. 27(5), 840-851, (2020).
[8] C. Ho, L. Morsut, Novel Synthetic Biology Approaches for Developmental Systems. Stem Cell Reports. 16(5), 1051-1064 (2021).
[9] L. Morsut, et.al., Engineering customized cell sensing and response behaviors using synthetic notch receptors. Cell, 164(4), 780-791 (2016).
[10] J.A. Thomson, et.al., Embryonic stem cell lines derived from human blastocysts. Science. 282(5391), 1145-1147, (1998).
[11] J. C. Lee, et.al., Instructional materials that control cellular activity through synthetic notch receptors. Biomaterials, 297, 122099. (2023).
[12] R. Kopan, M. X. Ilagan, The Canonical Notch signaling pathway: Unfolding the Activation Mechanism. Cell, 137(2), 216-233 (2009).
[13] L. Liu, A. Warmflash, Self-organized signaling in stem cell models of embryos. Stem Cell Reports. 16(5), 1065-1077 (2021).
[14] A. Deglincerti, et.al., Self-organization of human embryonic stem cells on micropatterns. Nature Protocols. 11(11), 2223-2232 (2016).
[15] E. Markham, Sleeping beauty awakens for Genome Engineering. Addgene blog. (2015).
[16] P. J. Wrighton, et.al., Signals from the surface modulate differentiation of human pluripotent stem cells through glycosaminoglycans and Integrins. Proceedings of the National Academy of Sciences of the United States of America, 111(51), 18126-18131 (2014).
[17] J. R. Klim, L. Li, P. J. Wrighton, M. S. Piekarczyk, L. L. Kiessling, A defined glycosaminoglycan-binding substratum for human pluripotent stem cells. Nature Methods. 7(12), 989-994 (2010).
[18] J. Davies, M. Levin, Synthetic morphology with agential materials. Nature Reviews Bioengineering. 1, 46-59 (2023).
Posted by buchanle on Tuesday, April 30, 2024 in May 2024.
Tags: bioactive materials, gastrulation, synthetic biology, Synthetic development, Tissue Engineering, WNT signaling