Synthesis and Characterization of Macromolecular Polymeric STING Agonists for Cancer Immunotherapy Applications
ABSTRACT
Cancer immunotherapy has revolutionized treatments for many cancers. One immunotherapy strategy is to utilize small molecule innate immune agonists to stimulate a response against cancer. Unfortunately, this strategy suffers limitations due to toxicity and poor pharmacokinetics. Precious research shows designing macromolecular STING (stimulator of interferon genes) agonists is an effective cancer immunotherapy method that improves characteristics that drugs alone don’t offer. STING agonists can be incorporated into polymeric carriers and lead to pro-inflammatory signaling to eliminate cancer cells. After creating these polymers and dosing cells, the immune stimulation of the polymers was evaluated to the agonist alone. DiABZI STING agonist polymeric constructs showed equivalent maximal IRF (interferon regulatory factors) signaling compared to free agonists. In addition, micelles were created with MSA-2 polymers that had cleavable linkers to release MSA-2 with or without a pH-responsive element. After assembling these particles, a stability study was done to analyze the morphology and disassembly of MSA-2 nanoparticles. Results found that the MSA-2 nanoparticles with a pH-responsive element showed a significant size change when challenged with dithiothreitol (DTT). The goal of this research was to identify which agonist performs best to improve upon limitations seen in other studies. In this study data was collected and analyzed in hopes to build upon what has been found in previous cancer immunology studies.
INTRODUCTION.
Thousands of diseases exist but one of the deadliest is cancer. Cancer occurs in the body due to uncontrollable cell division caused when natural controlling mechanisms (e.g., cell cycle checkpoints) become compromised. It can also spread to other tissues within the body and quickly become invasive. Many types of cancer therapies exist such as chemotherapy, radiation, and surgery but they can cause off-target toxicity, are invasive and can lead to other major complications. An appealing alternative is to utilize immunotherapeutic strategies to treat cancer which can reduce the side effects caused by other cancer treatments, utilizing the exquisite specificity and strength of the immune system to eliminate cancer, and providing long-lived immunological memory to prevent the recurrence of cancer. In order for these immunotherapies to work and efficiently eliminate the cancer, they require proper drug delivery systems.
Polymeric carriers have been studied for the purpose of drug delivery, in which they can solubilize hydrophobic drugs, increase blood circulation, preferentially drain to tumors due to the enhanced permeability and retention (EPR) effect, as well as have tune-ability in drug release based on linker choice. This research focuses on polymers because their size and weight can be modified and allow for controlled drug loading. The stimulator of interferon genes (STING) pathway is one natural innate immune pathway that can trigger potent anti-tumor innate immune responses and promote antigen presenting cell (APC)-mediated activation of T-cells [1]. Natural agonists for STING include cyclic guanosine monophosphate–adenosine monophosphate (cGAMP) and other cyclic dinucleotides (CDNs), [1]. Researchers have been steering away from these natural agonists because of their low activation rate and short half-lives. These receptors can also be bound with a synthetic agonist (MSA-2 or diABZI for STING) which leads to the activation of transcription factors, IRF, in the nucleus. STING predominantly results in IRF. Transcription factors transcribe genes that lead to the production of cytokines or type 1 IFNs (interferons) whose main purpose is to act as a pro-inflammatory signal to surrounding cells. T-cells can respond to this signal and plan their defense accordingly, with the aid of type 1 helper T (Th1) cells that facilitate extra activation of dendritic cells (DC) and natural killer (NK) cells.
Research in immunotherapy has been crucial to the development of safe, specific, and stable cancer treatments. In vitro and in vivo studies have been done to analyze the effectiveness of STING agonists paired with polymeric carriers [2]. Studies show larger polymer-agonist conjugates allow for more activation of STING, however, creating these conjugates has proven to be difficult [2]. The main reasoning behind finding a better delivery method is to improve the poor pharmacokinetics of small molecule, synthetic STING agonists, as well as to induce inflammation for more effective tumor elimination. Other major issues are encountered while studying immunotherapy such as the hydrophobicity of the agonists themselves and varying sizes that cause biostability issues in the body [3]. Polymer based CDN delivery systems are being researched using many different polymeric materials and proteins to provide the desirable shape, size and strength to deliver cancer treatment to T-cells, DCs, NK cells, APCs, and macrophages. Pre-clinical and clinical trials have been performed with STING agonist polymers as well as with antibodies to amplify the immune response to cancer [3]. Many issues can occur on the way to the tumor which is why the research behind polymeric drug carriers is so crucial to improving cancer treatment.
STING agonists show great potential in cancer immunotherapy and, when paired with polymeric carriers, this potential is significantly heightened. Finding the polymer that delivers treatment the best as well as making the best synthetic agonist for STING pathways is the main goal of the research lab I worked in. Our hypothesis is that designing macromolecular STING agonists will be an effective method for cancer immunotherapy and will improve characteristics that free drugs do not offer (e.g., safety, half life, pharmacokinetics, etc.). The main aim of this project is to improve current cancer treatments that show a significant amount of toxicity, as well as discomfort and pain for patients. The short half-life of natural agonists causes less activation of the transcription factors needed to provide adequate signals for T-cells, causing little to no tumor elimination [4]. Natural agonists also have subpar pharmacokinetics meaning that the drugs are poorly dispersed and don’t travel well throughout the body, polymers can be the solution to this because they can be easily modified to be tumor specific allowing for direct drug distribution [5]. These polymeric systems allow for the drug to make it through the circulating system and conclude in the spleen and liver. Small drugs have shown to get excreted through the kidney, so in turn, a bigger molecule will prevent this. The question we seek to answer is: How can polymeric carriers containing synthetic agonists such as MSA-2 and diABZI, improve activation of the STING immune pathway? The more specific question I focused my research on was: Which agonist led to the comparable immunostimulatory activity relative to the free agonist, so that it could be translated to in vivo cancer immunotherapy models?
MATERIALS AND METHODS.
In this project, we utilized RAFT polymerization, nanoparticle synthesis, and reporter cell lines to understand the structure-function relationship between free STING agonists and polymeric agonist constructs. Many different techniques can be used and are used, most showing similar results [3]. To best use resources available and collect the most accurate results, these processes were chosen.
RAFT Polymerization Process.
In order to analyze the effectiveness of STING polymers, the polymers were first synthesized. Reversible addition fragmentation chain transfer or RAFT is a polymerization process that allows for the conjugation of different monomers to the chain transfer agent (CTA) and creates the polymer. A CTA, monomer(s), and an initiator were used in all polymers, where the components varied based on the type of polymer being created. An ECT-based CTA (e.g., ECT, diABZI-Alkane-ECT, diABZI-ECT, or diABZI-DS-ECT) acts as the base of the polymer and allows for incorporation of the solubilizing monomers. ECT or ethylsulfanylthiocarbonyl, is a common agent utilized in RAFT polymerizations. The solubilizing monomers used in the polymers were dimethylacrylamide (DMA) or polyethylene glycol methyl ether (PEGMA), and the RAFT solvent was dimethylformamide (DMF). To begin, the RAFT inhibitors in DMA, PEGMA, and DMF were filtered out using a column filled with activated alumina. A thermally decomposing initiator was added in order for the reaction to take place and most polymerizations utilized a 5:1 CTA to initiator ratio (either V70 or AIBN was used for polymerizations depending on the monomer). The amount of DMF added was calculated to yield a 30% w/v percent for the reaction, which was performed in a 5- or 10-ml pear-shaped flask under inert conditions. Once all components were in the pear-shaped flask, it was put on a magnetic stir plate with a stir bar inside to ensure combination of all components. After 10 minutes of mixing a t (0) sample is taken to the NMR facility to be characterized. The vial was then wrapped in parafilm and a copper wire along with a septum. Purging the reaction solution displaces atmospheric oxygen (which quenches RAFT reactions) from the vial and replaces it with argon. Briefly, the flask was placed back on the stir plate to stir, a “spout” needle was inserted through the seal for oxygen displacement and a needle connected to an argon tank was inserted to the bottom of the flask. The flask was then left to purge for 10 minutes. After stirring and purging, the needles were removed from the flask, the flask was removed from the stir plate and placed in an oil bath set at either 40°C or 70° C depending on the type of initiator used. The reaction begins when the initiator is brought to its specified temperature, where the initiator decomposes generating radicals that lead to the formation of the polymer (Figure 1). After sitting in the oil bath for 24 hours a t(x) sample was taken and set aside for NMR (nuclear magnetic resonance). The polymer was then transferred to a dialysis membrane (with 2 kDa, 3.5 kDa, or 10 kDa molecular weight cut offs depending on the size of the polymer), along with DCM (dichloromethyane) and methanol. It was then dialyzed in a 1:1 ratio of DCM and methanol followed by acetone and then water, which purified out unreacted monomer(s) and other contaminants. After dialysis, the polymer was transferred to a 50 mL conical tube, put in the freezer at -80°C overnight, and then placed on the lyophilizer to get rid of water through sublimation. After lyophilizing for 3 days, the polymer could then be used. Activation of targeted pathways as a function of agonist concentration was analyzed for all polymers with respect to free agonist controls.

Nuclear Magnetic Resonance.
An H-NMR (Hydrogen Nuclear Magnetic Resonance) machine is used to detect hydrogen in a sample and determine conversion of monomers. A t(0) was taken before polymerization, and a t(x) was taken after polymerization, then analyzed to find conversion rate, as well as valency, the number of agonists or monomers on the polymer. The t(0) is expected to show hydrogen peaks from unpolymerized DMA, while a t(x), which is after polymerization, it is expected to show a large decrease in unpolymerized DMA peaks and broadening of peaks in the low regions due to incorporation into the polymer. To find the percentage of conversion for a particular polymer Eq. 1 is used where uDMA is an unpolymerized DMA peak and pDMA is a polymerized DMA peak.
\[\frac{uDMA\ Peak – pDMA\ Peak}{uDMA\ Peak}=\% Conversion\tag{1}\]
Dose Response.
In order to test if the polymer adequately activated the STING pathway, cells were dosed with the polymer and other control treatments. In a 96 well cell culture plate 100 µL of cells were added to the innermost 60 wells, 200 µL of PBS was added to the outer wells to prevent evaporation of inner wells. Stock solutions of the treatments were made in DMSO (dimethyl sulfoxide) and serially diluted in media at indicated dosages. Negative control wells were filled with an equal percentage of DMSO as the highest dosed treatment groups. Then, 100 µL of treatments were then added to the appropriate inner wells of the plate.
Quanti-Luc.
Quanti-Luc is an agent used to quantify STING activation in the cells after being dosed with treatments. It quantifies secreted Lucia luciferase which indicates STING activation. Cells were spun down after 20-hour treatment, incubated for 5 min at 1500 rpm. The auto injector of the Synergy plate reader was primed with 1000 µL of DI water, twice, followed with two priming cycles using 500 µL of the Quanti-Luc reagent. 20 µL of media from treated cells was then added to a white 96 well plate in the dark and placed in the plate reader, which injected 50 µL of Quanti-Luc per well. Luminescence was then read using the Quanti Luc auto injector plate reader setting.
Nanoparticles.
The main purpose of a nanoparticle is to improve half-life and cellular uptake. A micelle is a type of self-assembling nanoparticle that has a hydrophilic and hydrophobic component that reacts when in contact with water. The hydrophilic component surrounds the dense, hydrophobic core to form the micelle. To form micelles, a polymer was dissolved in DMSO at a 20 mg/ml concentration and then diluted 10x into deionized water. The solution was then vortexed for 20 seconds and left to sit for an hour before being prepared for the Zetasizer, which evaluates the particles size and dispersion. To prepare for the Zetasizer, 50 µL of the micelles were transferred to semi-micro cuvettes along with 1 mL of PBS.
Cell Culture.
THP-1 Dual (catalog: thpd-nfis) cells were used in this study. Cells were maintained based on InvivoGen’s standard manufacturer’s instructions.
RESULTS.
Many different agonists were used for the polymers such as diABZI or MSA-2. It was important for this project to examine the characteristics of the polymers. Different concentrations and weights of the agonists were aimed for and tested to determine the actual concentration of agonists that ended up in the polymer, (valency). Polymers made with diABZI had higher conversion rates than those made with MSA-2 (Table 1).
Table 1. Chart displaying contents and final polymers after RAFT polymerization. All polymers were synthesized using an ECT-based chain transfer agent. The general polymer nomenclature scheme describes if the ECT was modified with another molecule (e.g., PEG-2k-ECT is named PEG-2k, whereas a polymer made with ECT would be left blank) followed by the monomer composition of the polymer (e.g., a MSA-2 and PEGMA copolymer is named p(MSA-2-co-PEGMA)). | ||||
Agonists | Conversion | Target MW (kDa) | Actual MW (kDa) | Agonist Valency |
P1: p(MSA-2-co-PEGMA) | MSA-2: 100% PEGMA: 77.8% | 25.9 | 21.1 | 8 |
P2: p(MSA-2-co-PEGMA) | MSA-2: 88.3% PEGMA: 88.3% | 51.3 | 45.2 | 26.3 |
P3: p(mSA-2) | MSA-2: 79.2% | 12.8 | 10.1 | 19.6 |
P4: PEG-2k-p(MSA-2) | MSA-2: 77.5% | 10.2 | 8.2 | 6.8 |
P5: PEG-2k-p(MSA-2-co-DEAEMA) | MSA-2: 79.2% DEAEMA: 74.0% | 10.5 | 8.6 | 9.6 |
P6: Short diABZI-Alkane-P(DMA) | DMA: 99.2% | 23.0 | 22.8 | 1 |
P7: Medium diABZI-Alkane-p(DMA) | DMA: 98.1% | 53.0 | 52 | 1 |
P8: Long diABZI-Alkane-p(DMA) | DMA: 99.3% | 175.2 | 174.0 | 1 |
P9: Short diABZI-p(DMA) | DMA: 99.5% | 25.7 | 25.6 | 1 |
P10: Medium diABZI-p(DMA) | DMA: 97.4% | 54.9 | 53.5 | 1 |
P11: Long diABZI-p(DMA) | DMA: 93.5% | 175.1 | 163.7 | 1 |
P12: Medium diABZI-DS-p(DMA) | DMA: 100% | 50.1 | 50.1 | 1 |
Short, medium and long polymers are differentiated by their molecular weight, which is changed based on how much monomer is added relative to the CTA. After dosing cells, Lucia luciferase in the supernatant was quantified with Quanti-Luc, respectively. p(MSA-2-co-PEGMA) showed minimal activation when compared to the MSA-2 agonist alone, while PEG-2k-p(MSA-2) NPs on the other hand showed no activation at all (Figure 2A). In contrast, short, medium, and long diABZI-Alkane-p(DMA) show similar maximal activation when compared to the diABZI-Alkane-ECT alone (Figure 2B). Short, medium, and long diABZI-p(DMA) also show similar performance when compared to the diABZI-ECT and diABZI alone as well as when compared to a diABZI-NH2, which is the parent compound for ECT-based diABZI molecules (Figure 2C). A comparison of two of the same medium diABZI-p(DMA) polymers was done, one with a disulfide bond and one without. Results show similar STING activation of the polymers and CTAs (Figure 2D).
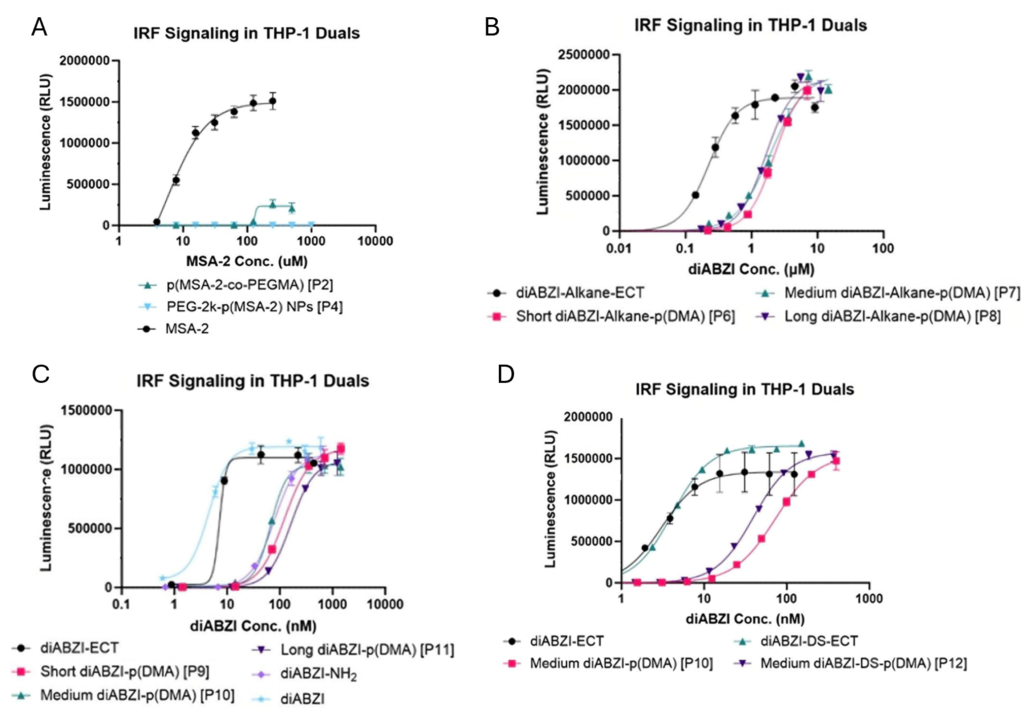
Results regarding the size of MSA-2 nanoparticles show a mono-disperse size. Previous studies show that the particles distribute contents best around 100 nm in size [3]. Results found in this study show that MSA-2 nanoparticles are dispersed averaging around 115 nm with normal distribution (Figure 3A). After the MSA-2 NPs with a pH responsive element were challenged with a disulfide breaking element (DTT), it is observed that they have a multimodal distribution (Figure 3B).
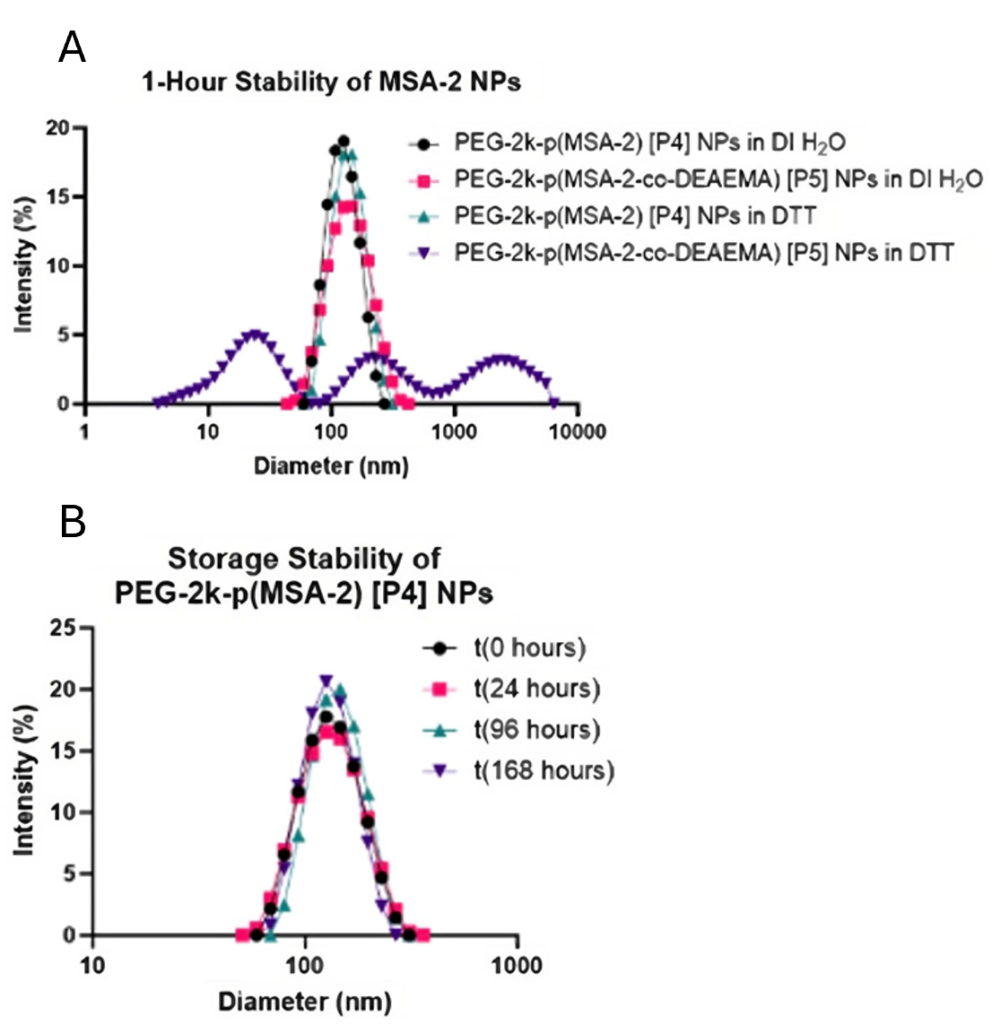
DISCUSSION.
Current technologies used for cancer immunotherapy show that polymers used for STING activation improve issues surrounding polymeric drug distribution [5]. Many experiments were performed in order to find the polymer that best fits both the needs of the drug being delivered, and the amount of activation required to improve immune response.
Results accumulated in this study show polymers can cause signaling of the immune pathway just as well as the agonist alone. Results also show significant activation of immune response in THP-1 Dual cells (Figure 2A, B, C, D). DiABZI polymers show similar final activation when compared to the agonist alone. It is important to note the activation of the polymers compared to the concentration of the agonists. There is a similar amount of activation but at a greater concentration for the short, medium, and long polymers, however the concentration of the agonist is not as significant as the fact that it caused equal activation without all the toxic downsides seen by the agonist alone. To quantify the data, EC50 values (where the concentration is halfway between minimum and maximum) are compared using an ANOVA based theory that allows the assumption that any individual point at a certain drug concentration that does not have overlapping standard deviation bars with another point can be considered significantly different. This means that most of the polymers are significantly similar to each other but significantly different from their agonist counterparts, because error bars aren’t overlapping. The concentration at which the activation begins to stabilize is where the least appreciable cell death occurs, and the least toxicity can be assumed.
The significance of this study is found within the polymeric makeups. A majority of the polymers used in dose responses were constructed using combinations of different agonists and CTA’s. Cancer therapies are still widely researched and studied to be improved and polymers have shown to be an effective way to control the immune response. This study provokes deeper research into more agonists that can be used in polymers as well as in nanoparticles.
Many limitations involved in the study involve impurities as well as shelf life. A similar limitation encountered was the impurity of polymers made, polymers are subject to degradation over time, while this is a small change in the accuracy or the polymer makeup it is a factor. Not knowing the reason for lack of activity has proved to be an issue, if we were able to pinpoint what was causing the deprivation, we could find a solution but since we cannot, we must rework the entire polymer. Future directions for polymeric drug delivery as a whole include the continuation of agonist containing polymer testing, as done in this study. Activation of the STING pathway using these polymers is ideal and using agonists such as MSA-2 and diABZI has shown to be an effective way to do so. By activating pathways using agonists to activate STING, and other pathways that allow for faster and more efficient drug delivery, it is possible to reduce the risks of current toxic cancer treatments. Polymeric nanoparticles used for cancer treatment allow for direct vaccine delivery for a safer small molecule cancer immunotherapy.
REFERENCES
- Smits E., Ponsaerts P., Berneman Z., Tendeloo V., The Use of TLR7 and TLR8 Ligands for the Enhancement of Cancer Immunotherapy, The Oncologist. 13, 859–875, (2008).
- Bhatnagar S., et.al, Combination of STING and TLR 7/8 Agonists as Vaccine Adjuvants for Cancer Immunotherapy, PubMed Central, 14, 6091, (2022).
- Su T., Zhang Y., Valerie K., Wang XY., Lin S., Zhu G. STING activation in cancer immunotherapy. Theranostics, 9, 7759-7771, (2020)
- Sellars M., Cancer Vaccines: Building a Bridge Over Troubled Waters, Cell. 185, 2270-2788, (2022).
- Shae D., Baljon J., Palmen M., ect. Co-delivery of Peptide Neoantigens and Stimulator of Interferon Genes Agonists Enhances Response to Cancer Vaccines, ACS NANO. 8, 9904-9916, (2020).
- Dragojevic J., Ryu J., Raucher D., Polymer-Based Prodrugs: Improving Tumor Targeting and the Solubility of Small Molecule Drugs in Cancer Therapy, Molecules, 20, 21750–21769, (2015).
Posted by buchanle on Tuesday, April 30, 2024 in May 2024.
Tags: Agonist, Immunotherapy, Nanoparticle, polymer