Deciphering the Impact of Cytomegalovirus on Endothelial Cell Function and Endothelial Nitric Oxide Synthase Expression
ABSTRACT
This study aims to investigate the potential contribution of cytomegalovirus (CMV) to the pathogenesis of cardiovascular disease (CVD), focusing on its effects on endothelial cell movement and endothelial nitric oxide synthase (eNOS) expression. Ex-vivo experiments were conducted using primary human arterial endothelial cells (HAECs). Time-lapse imaging was used to track movement in the presence of metabolic pathway inhibitors (glucose and oxidative phosphorylation) and after infection with recombinant CMV-expressing red fluorescent proteins (RFP). Recombinant CMV.RFP significantly increased eNOS expression in HAECs. Moreover, cell tracking analysis demonstrated decreased total distance traveled after infection with CMV compared to uninfected cells over 24hrs. However, all other aspects of cell movement were unchanged by CMV infection. In conclusion, an increase in eNOS may indicate oxidative stress mechanisms are activated, contributing to endothelial dysfunction manifested by decreased movement, which may be important in endothelial homeostasis. While this study used one laboratory strain of CMV, additional studies of molecular pathways using different CMV strains may provide insights into the role of CMV in the pathophysiology of CVD. Further research will elucidate the underlying mechanisms and potential therapeutic strategies for targeting CMV-associated endothelial dysfunction.
INTRODUCTION.
Cardiovascular disease (CVD) is the primary cause of mortality worldwide [1]. CVD includes various heart and blood vessel conditions such as coronary artery disease, high blood pressure, stroke, and heart failure. Endothelial cells, responsible for regulating communication between the bloodstream and surrounding tissues, are critical in maintaining vascular health and function [2]. Individuals living with Human Immunodeficiency Virus (HIV), referred to here as Persons with HIV (PWH), are particularly susceptible to endothelial dysfunction, which significantly amplifies the risk of CVD [3]. While several mechanisms are thought to be important, cytomegalovirus (CMV) coinfection in PWH is thought to contribute to high morbidity and mortality from different comorbid diseases including CVD [4].
CMV infection is highly prevalent in the United States, with a significant proportion of the population having been infected by the age of 5, and over half of adults infected by the age of 40 [5]. CMV primarily targets endothelial cells, macrophages, and T lymphocytes, making it an interesting virus for studying its effects on endothelial and cardiovascular function [6]. HIV is a virus that attacks the immune system, weakening the body’s ability to fight off infections and diseases such as CMV. Understanding the impact of CMV infection on endothelial cell function may provide valuable insights into the mechanisms underlying CMV-associated cardiovascular complications. We hypothesize that CMV infection induces alterations in the function of endothelial cells which could lead to increased inflammation within blood vessels due to the increased recruitment of immune cells to medium and large coronary arteries and increased atherosclerosis [7].
Endothelial nitric oxide synthase (eNOS) is responsible for the production of nitric oxide (NO), a potent vasodilator that plays a pivotal role in maintaining vascular homeostasis. Endothelial dysfunction is characterized by a reduction in NO availability within blood vessel walls, leading to impaired vasodilation and compromised regulation of blood flow [8]. Endothelial dysfunction is associated with various symptoms and manifestations of CVD. This study aims to investigate the potential contribution of cytomegalovirus (CMV) infection to the pathogenesis of CVD, with a specific focus on its impact on endothelial function and eNOS expression.
This project used human aortic endothelial cells (HAECs) to study the perturbed responses after infection with CMV. Specifically, we used time-lapse imaging to study endothelial cell movement, proliferation, and eNOS expression after infection with CMV. Investigating these changes will contribute to the understanding of CMV-mediated changed in endothelial function and its potential role in the development and progression of CVD. Furthermore, elucidating the mechanisms by which CMV affects endothelial cells may aid in the development of targeted therapeutic interventions aimed at preventing or mitigating CMV-associated cardiovascular complications. The results obtained from this research may provide valuable insights into the interplay between CMV infection and endothelial dysfunction, ultimately paving the way for novel strategies for the prevention and treatment of CMV-associated cardiovascular complications.
MATERIALS AND METHODS.
Cell Culture.
HAECs were obtained from Lonza (Catalog #CC-2535) and cultured in vascular growth media supplemented with 10% fetal bovine serum (FBS: Atlas Biologicals, Catalog #F-0500-D) and endothelial growth factors (GM-2 Endothelial Cell Growth Medium-2 BulletKit, Catalog #: CC-3162).
Recombinant CMV.
We received recombinant CMV strain AD169 as a gift to the lab from Dr. Eain Murphy (Syracuse-Upstate Medical University) [9]. This recombinant virus expresses the red fluorescence protein referred to as CMV.RFP.
CMV Infection.
For cell tracking, HAECs were infected with CMV.RFP at a multiplicity of infection (MOI) of 1.
Western Blot.
Approximately 400,000 uninfected HAECs and 400,000 CMV-infected HAECs (MOI of 0.1 and 1) were lysed using RIPA buffer for the first experiment. In the second experiment, confluent 6-well plates were infected with low and high titer CMV virus (~ MOI 0.1 and 1). The cell lysates were run on an 8% denaturing gel and proteins were separated by electrophoresis. Ponceau Stain was used to estimate the total amount of proteins in each well loaded with cell lysate. An eNOS-140kD antibody (Cell Signaling Technology, Catalog #32027S) was used to detect the eNOS protein, phospho-eNOS was detected using (Cell Signaling Technology, Catalog #9571S), and iNOS (Cell Signaling Technology, Catalog #20609S). To quantify the protein, an antibody against glyceraldehyde-3-phosphate dehydrogenase (GAPDH, 37kD), an enzyme involved in glycolysis and cellular energy production, was used as a loading control. The relative expression of eNOS was calculated by adjusting for the amount of protein loaded in each well using GAPDH as a control.
Metabolism Assays.
Non-confluent HAECs were cultured in media only and imaged using the EVOS M5000. To study the effects of different metabolic pathways, we incubated the HAECs with glucose inhibitors, 100 mM 2 Deoxy-D-Glucose (2DG), 1.5 μM oligomycin, or a combination of both 2DG and oligomycin. HAECs were imaged after the addition of the inhibitors for approximately 1 hour. Cell tracking was performed as below.
Transmission Electron Microscopy.
Endothelial cells were fixed with 2.5% glutaraldehyde solution in 0.1 M sodium cacodylate buffer. After secondary fixation, samples were washed for five minutes with 0.1 M sodium cacodylate buffer (7.3 pH) followed by two five-minute washes with diH2O. The samples were then incubated with 2.5% uranyl acetate, diluted with H2O, at 4 °C overnight. The cells were then dehydrated using an ethanol gradient series. The ethanol was then replaced with Eponate 12™ mixed in 100% ethanol in a 1:1 solution, at room temperature for 30 mins. This was repeated three times for 1 hour using 100% Eponate 12™. The plates were cured in an oven at 70 °C overnight. The plates were cracked upon hardening, and the cells were separated by submerging the plate in liquid nitrogen. Images were acquired by TEM on either a JEOL JEM-1230, operating at 120 kV, or a JEOL 1400, operating at 80 kV. 78.
Tracking of Cell Movement.
HAECs were cultured in 6-well plates at a density of approximately 60,000 cells per well. The cells were maintained in a tissue culture incubator at 37°C with 5% CO2. After infection, the HAECs were imaged over 24 hours using the EVOS M5000 imaging system and on-stage incubator with supplemental CO2. Individual images captured every 5 minutes over 24 hours were stacked using the Fiji (ImageJ) software. HAECs in the first frame of the time-lapse were tracked using the Manual Tracking plugin. We recorded both the x and y coordinates and frames of each cell over time. From the tracking data, we calculated the total distance traveled, net distance traveled, persistence (duration of movement in a single direction), and the rate of movement for each cell.
Statistical Analysis.
We used Mann-Whitney non-parametric t-tests to compare the total distance, net distance, persistence, and rate between the two cell types. Radial plots were generated to illustrate cell movement patterns, and violin plots with t-test values were created to visualize the differences between cell types. Statistical analysis was performed using GraphPad Prism version 9.5.0.
RESULTS.
Human arterial endothelial cells are highly dynamic cells that depend on both glucose and oxidative phosphorylation for movement in cell culture.
It is important to measure the reliance of HAECs on glycolysis and oxidative phosphorylation to understand the metabolic pathways underlying CVD and to show the cells using energy to function. Endothelial cells depend on migration for many of the functions to maintain vessel integrity, which has implications for the development of cardiovascular disease [10, 11]. While endothelial migration is complex and depends on several different cues in vivo, endothelial cells in culture migrate until the cells reach confluency. We used endothelial cell movement in culture to study endothelial function in the setting of CMV infection. When HAECs were incubated with a specific glucose inhibitor, 2DG, there was a significant reduction in the movement (Figure 1A). A similar reduction was seen when we inhibited the oxidative phosphorylation pathway and combined (Figure 1A). The total distance and net distance traveled were significantly lower with each of the inhibitors compared to the no-inhibitor control (Figure 1C-D). While inhibiting both the glucose pathway and oxidative phosphorylation was additive, there was no significant difference in the reliance of HAECs on glucose versus oxidative phosphorylation. We also quantified HAEC cell persistence, defined as the tendency of the cells to move in the same direction. While not statistically different, HAECs had higher persistence when inhibited by 2DG (p=0.07) and oligomycin (p=0.08) compared to uninhibited cells which move more freely (Figure 1E). The rate of movement was also significantly decreased when glycolysis and oxidative phosphorylation were inhibited (Figure 1F).

Human CMV infects HAECs and paradoxically leads to an increase in eNOS.
To establish whether human arterial endothelial cells can be infected by recombinant CMV in culture, HAECs were infected with recombinant CMV that expresses red fluorescent protein (RFP) for 24 hours. A productive infection with viral replication was confirmed by the expression of red fluorescence in the infected compared to uninfected HAECs (Figure 2A-B). One of the important functions of endothelial cells is the regulation of blood pressure which is mediated by the expression of nitric oxide. Endothelial nitric oxide synthase (eNOS) is an enzyme that produces the nitric oxide expressed by endothelial cells. Interestingly, endothelial cells, when infected with CMV at an MOI of 1 expressed higher levels of eNOS compared to those infected at an MOI of 0.1. This was indicated by an increase in the eNOS band intensity at the higher MOI (Figure 2C) and confirmed by the normalized expression signal of eNOS that was significantly higher compared to uninfected endothelial cells (p<0.05). While eNOS expression is beneficial, excessive amounts of eNOS can be harmful leading to cellular damage due to nitric oxide reacting with other molecules expressed in the endothelial cells. In Figure 2C, the eNOS band intensity is increased with CMV MOI 1, while in Figure 2D, the total eNOS is higher for the lower dose CMV, which is interesting. The Ponceau Stain, which shows the total amount of proteins in each sample was similar between uninfected and infected endothelial cells. Another control, GAPDH, indicates an equal amount of protein was loaded for samples and controls. The infection of HAECs with CMV was repeated at a low and high multiplicity of infection. Although there were fewer samples, eNOS increased with infection compared to uninfected control (Figure 2D). Phospho eNOS and iNOS also trended towards higher levels after infection but this was not statistically significant.
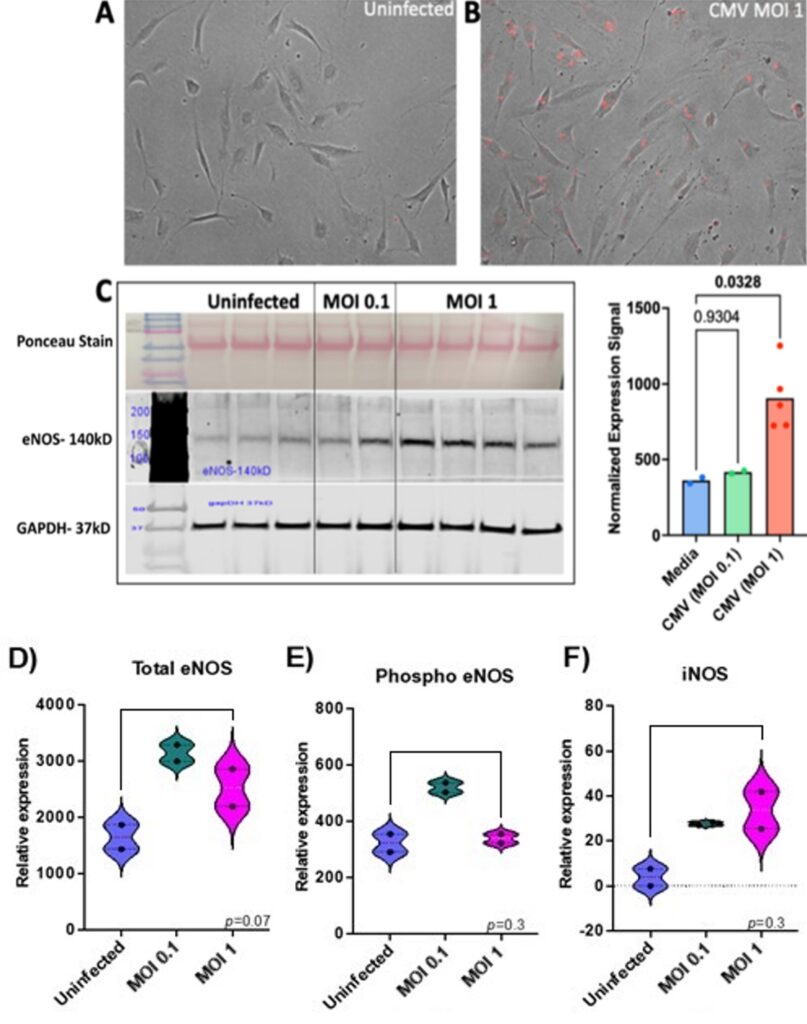
Human arterial endothelial cells infected with CMV remain highly dynamic during the early phase of infection.
Endothelial cells are highly dynamic, and movement is important for their normal function including wound healing and angiogenesis. While cell cultures are a simplified model to understand the effect of CMV on endothelial function, pairing culture with time-lapse imaging allows for the study of changes in cell movement because of infection. We traced the movement of uninfected cells (not shown) and CMV-infected HAECs (Figure 3A). The red fluorescence shows infected cells, and the blue circles highlight multi-nucleated HAECs and their change over time to illustrate their movement (Figure 3A). The radial plot is used to illustrate the movement of each tracked cell within the field of view of the microscope at the time of imaging (Figure 3B). This radial plot shows the sparse and wide range of movement patterns of the endothelial cells in culture, and the individual tracks within their different paths of movement (Figure 3C). We captured HAECs infected with CMV dividing into two daughter cells, with each daughter cell remaining infected. This is important because although there’s no evidence showing that endothelial cells directly infect other cells with viruses, it is demonstrated that they can divide, giving rise to infected daughter cells in culture.
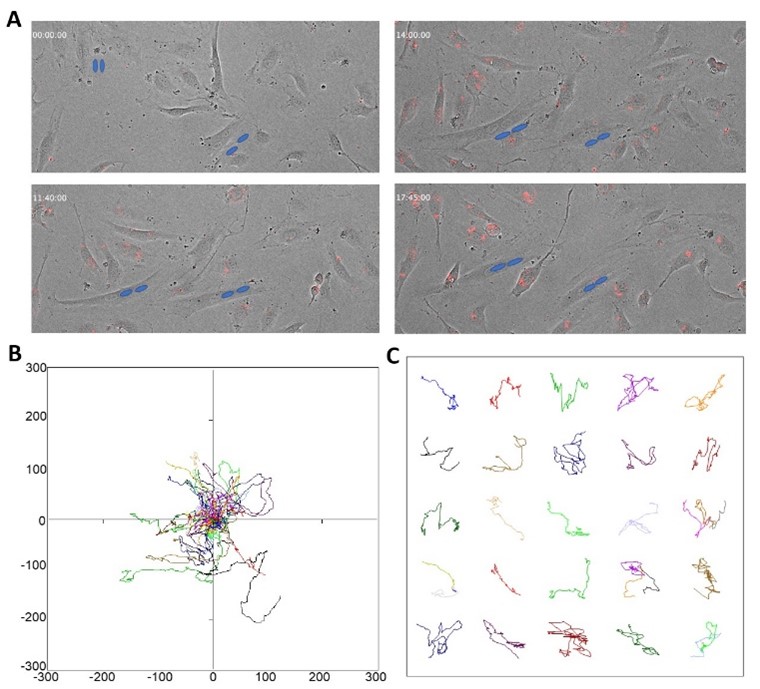
DISCUSSION.
In this study, we observed that primary human arterial endothelial cells were susceptible to infection with CMV.RFP. We tracked infected endothelial cells over a 24-hour window and showed that acutely, endothelial cells remained dynamic, as captured by their motility. At a molecular level, CMV infection increased eNOS expression with a minimal but measurable increase in iNOS. Nitric oxide, produced by eNOS, is a critical vasodilator with a pivotal role in vascular homeostasis. Notably, eNOS expression may be negatively correlated with endothelial cell proliferation and migration [12]. Therefore, in our in vitro assays, the unexpected increase in eNOS with CMV infection may offer an advantage to the virus and may be influenced by viral proteins that are expressed during an acute infection. This may represent an adaptive mechanism that promotes eNOS expression in acute infection that allows for the virus to replicate and infect more cells, and perhaps another host before the immune system is triggered to clear the virus. Based on previous publications, while eNOS expression may be increased, this could be uncoupled with an increase in the production of superoxide leading to endothelial dysfunction [13]. HIV infection is associated with elevated levels of asymmetric dimethylarginine (ADMA) compared to HIV-negative individuals [14]. ADMA is an endogenous protein that inhibits eNOS and is associated with endothelial dysfunction. While in culture, ADMA may not be an important driver of the differences, the importance of eNOS uncoupling in the contribution of endothelial dysfunction despite an increase in eNOS expression needs to be further investigated and could be explained by increased ADMA expression levels with HIV.
The dynamic nature of endothelial cells relies on both glucose and oxidative phosphorylation. This goes along with the numerous numbers of mitochondria present in the endothelial cells as evidenced by TEM imaging. Mitochondrial fusion mediated by the virus, to allow for the CMV virus to effectively replicate in endothelial cells, may in the long run affect other energy requiring cellular functions that further contribute to endothelial dysfunction [15]. Future studies will investigate whether targeting metabolic pathways may slow down CMV infection and improve endothelial cell function. Additionally, it will be important to measure if NO levels are really increased in CMV-infected cells, as there may be increased abundance of eNOS, but not functional increase of enzyme activity.
CONCLUSION.
Our study provides evidence of CMV-induced alterations in endothelial cell function, characterized by increased eNOS expression and effects on motility dynamics. These findings highlight the potential role of CMV in endothelial dysfunction, which is a key contributor to cardiovascular diseases. While this study presents a significant step in understanding the mechanisms of how CMV impacts endothelial cells, further research is needed to explore the long-term consequences of CMV infection and its interactions with immune cells. Continued investigation may uncover strategies for the prevention and treatment of CMV-associated cardiovascular complications, ultimately advancing the understanding of CVD pathogenesis, and placing us on a path toward better treatment of CMV-associated cardiovascular complications and subsequently, better patient outcomes. Future, research will continue to examine the interaction between CMV-infected endothelial cells and other immune cells, such as monocytes, as these interactions are believed to play a crucial role in the development of cardiovascular disease. Understanding the interplay between immune cells and infected endothelial cells may provide further insights into the mechanisms underlying CMV-associated cardiovascular complications.
ACKNOWLEDGMENTS.
I would like to thank the SSMV and my advisor, Dr. Angela Eeds, for this research opportunity. I would also like to thank Dr. Celestine Wanjalla and the Wanjalla Lab for their mentorship and support throughout the process. Supported by Doris Duke CSDA 2021193 (CNW), K23 HL156759 (CNW), Burroughs Wellcome Fund 1021480 (CNW).
REFERENCES.
- A. S. V. Shah et al., Global Burden of Atherosclerotic Cardiovascular Disease in People Living With HIV. Circulation. 138, 1100–1112 (2018).
- K.-H. Park, W. J. Park, Endothelial Dysfunction: Clinical Implications in Cardiovascular Disease and Therapeutic Approaches. Journal of Korean Medical Science. 30, 1213 (2015).
- J. H. Stein et al., Effects of HIV Infection on Arterial Endothelial Function. Arteriosclerosis, Thrombosis, and Vascular Biology. 41 (2020).
- M. Lichtner et al., ICONA Foundation Study, Cytomegalovirus coinfection is associated with an increased risk of severe non-AIDS-defining events in a large cohort of HIV-infected patients. The Journal of infectious diseases. 211, 178–86 (2015).
- S. A. S. Staras et al., Seroprevalence of Cytomegalovirus Infection in the United States, 1988-1994. Clinical Infectious Diseases. 43, 1143–1151 (2006).
- M. A. Jarvis, J. A. Nelson, Human cytomegalovirus persistence and latency in endothelial cells and macrophages. Current Opinion in Microbiology. 5, 403–407 (2002).
- A. Al-Soudi, M. H. Kaaij, S. W. Tas, Endothelial cells: From innocent bystanders to active participants in immune responses. Autoimmunity Reviews. 16, 951–962 (2017).
- Förstermann, U., Münzel, T., Endothelial Nitric Oxide Synthase in Vascular Disease. Circulation. 113, 1708–1714 (2006).
- E. Murphy et al., Coding potential of laboratory and clinical strains of human cytomegalovirus. Proceedings of the National Academy of Sciences of the United States of America. 100, 14976–14981 (2003).
- U. R. Michaelis, Mechanisms of endothelial cell migration. Cellular and Molecular Life Sciences. 71, 4131–4148 (2014).
- L. Lamalice, F. Le Boeuf, J. Huot, Endothelial Cell Migration During Angiogenesis. Circulation Research. 100, 782–794 (2007).
- S. Bu et al., Endothelial cell-specific loss of eNOS differentially affects endothelial function. PLOS ONE. 17, e0274487–e0274487 (2022).
- I. L. Leskov, J. Whitsett, J. Vasquez-Vivar, K. Y. Stokes, NAD(P)H oxidase and eNOS play differential roles in cytomegalovirus infection-induced microvascular dysfunction. Free Radical Biology and Medicine. 51, 2300–2308 (2011).
- R. V. Parikh et al., Elevated levels of asymmetric dimethylarginine are associated with lower CD4+ count and higher viral load in HIV-infected individuals. Atherosclerosis. 229, 246–252 (2013).
- J. A. Combs et al., Human Cytomegalovirus Alters Host Cell Mitochondrial Function during Acute Infection. Journal of Virology. 94 (2020).
Posted by buchanle on Tuesday, April 30, 2024 in May 2024.
Tags: Cardiovascular, Cytomegalovirus, Endothelial, eNOS, HIV