Jackson Lab reveals relationship between transport proteins and brain disease
Most of us rely heavily on shipping services like FedEx or UPS to ensure we receive the correct packages. If that system was disrupted, parcels would end up lost or in the wrong place.
Similarly, all human cells require large protein coat complexes, working at transport hubs called endosomes, to coordinate the transport of fatty lipid and protein molecules required for human brain health. In new research conducted by Lauren Jackson’s Lab, scientists have uncovered how those individual proteins interact with one another, and how those interactions may cause brain disease.

Funded by the National Institutes of Health and led by Research Assistant Professor of Biological Sciences Mintu Chandra, the team analyzed the protein complex retromer and its interactions with sorting nexins (SNXs), which are large groups of proteins.
Retromer and specific SNXs work together to assemble structures with a fatty membrane, ensuring that molecules are delivered to the correct destination. If mutations were to occur, or retromer and SNXs were to go missing, then molecules would be incorrectly dispersed—a phenomenon linked to multiple neurodegenerative diseases, such as Alzheimer’s, Parkinson’s, and amyotrophic lateral sclerosis (ALS).
“This discovery provides fundamental insights into the molecular architecture of sorting nexin complexes, which play an essential role in maintain a stable cell environment,” Chandra said. “Given the pivotal functions of SNX and retromer in neuronal signaling and disease-associated pathways, our findings have significant implications for neurodegenerative disorders.”
Chandra, alongside Associate Professor of Biological Sciences Lauren Jackson, Jackson Lab Manager Amy Kendall, and Altos Labs Scientist Marijn Ford, combined biochemistry, biophysics, imaging, and AI-based computational modeling to demonstrate the protein interactions. They further analyzed how a specific sorting nexin, SNX27, interacts directly with VARP, another regulatory protein.
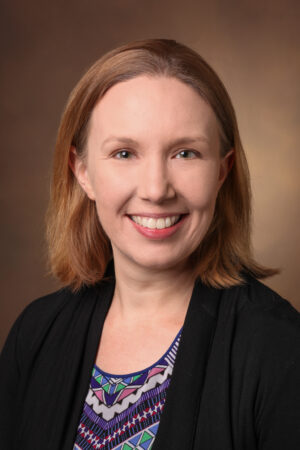
“These findings are important for us to understand why humans get brain disease and how we might treat it,” Jackson said. “One thing we learned from this study is that a couple of our proteins change shape when they interact with each other, so if we think medium- to long-term, it suggests we might want to examine how small molecules or drugs could be used to treat something like brain disease by locking a protein in an active or inactive state.”
Looking ahead, the team plans to investigate the structural organization of these large protein complexes in cells by combining ion beam milling with cryo-electron tomography—a technique that uses an electron microscope to create three-dimensional images of frozen biological samples.
“Additionally, we will explore how disruptions in this complex contribute to disease pathology, with the broader goal of identifying therapeutic strategies to restore endosomal function in neurodegenerative disorders,” Chandra said.
Read the full paper on ScienceAdvances.