Physics Students Win U.S. Department of Energy Research Fellowships
Two graduate students in the Department of Physics and Astronomy have been awarded coveted U.S. Department of Energy (DOE) fellowships to advance their research. David Curie, a third-year physics Ph.D. student, received an Office of Science Graduate Student Research (SCGSR) Fellowship to conduct part of his dissertation research in a DOE laboratory. Laura Nichols, a first-year physics Ph.D. student, received a Computational Science Graduate Fellowship (CSGF) in overall support of her dissertation research in computational physics.
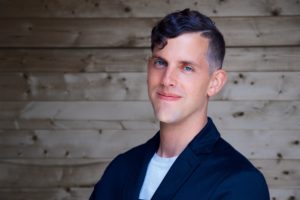
Curie’s work focuses on single-photon sources, which can be used in quantum communications and possibly quantum computing. Currently, fiber optics are the most common system for using light (photons) to transmit data. However, that data typically travels in streams of pulses, or bits, which hackers can intercept without leaving traces of interference. Single-photon sources can generate entangled photon pairs to use in place of traditional bits—an arrangement that allows for more secure communication protocols. To operate reliably, however, the best available single-photon sources often must be cooled to extreme temperatures. This requirement creates economic hurdles to widespread adoption of the technology. Enter hexagonal boron nitride (h-BN), a material that can be stripped down to two-dimensional thicknesses on the same scale as the nanomaterial graphene. H-BN can emit single photons at room temperature, but current h-BN emitters are not efficient enough to be reliable. Curie’s research seeks to improve the efficiency of h-BN emitters and make them a more economical alternative by engineering the environment around the material in a widely adoptable way.
Curie didn’t originally intend to study single-photon sources. In fact, he entered college planning to become a chemistry major. But after taking a physics class, he became interested in the subject and did research on lasers and atomic optics (the properties and behavior of light-matter interactions). That work led to post-baccalaureate research at Oak Ridge National Laboratory.
“An opportunity came up for a research project, and I happened to have some tangential skills,” Curie said. He decided to earn his Ph.D. at Vanderbilt partly because of the physics department’s relationship with Oak Ridge, and he’ll now return to that lab for his SCGSR fellowship.
An indirect descendant of renowned physicists Marie and Pierre Curie, David sees himself as both following in family footsteps and paving the way for the next generation. As a graduate teaching assistant, he often asks to be assigned to classes that attract students who are just fulfilling a requirement.
“People who aren’t interested in physics are probably the ones most worth reaching out to,” he said. “It’s so rewarding when I get them to like it. Ten years ago, I wouldn’t have thought, ‘I’m going to go work at a national lab and do something cool.’ But I had professors who were encouraging and emphasized that it’s not about being smart, it’s about being hard-working and diligent. It’s about whether you can reason through a problem and find out what you don’t know.”
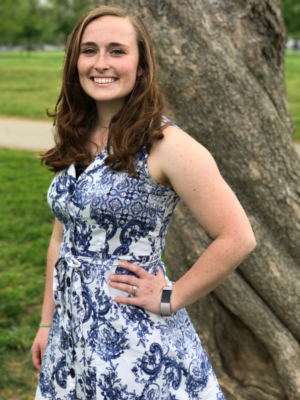
Reasoning through problems is also what attracted Laura Nichols to physics. The CSGF fellowship, awarded to only about 30 individuals nationally per year, supports Ph.D. candidates in the computational sciences: students who use computer programming to solve problems in scientific disciplines such as physics, biology, and chemistry. Nichols first encountered computational science as a high school student, when she attended the Tennessee Governor’s School for Computational Physics. She found the subject so interesting that she decided to study computational physics in college and earn her Ph.D. in the field. At Vanderbilt, she’s researching ways to predict how defects in materials might affect the performance of semiconductors.
Manufacturers place semiconductors in a host of everyday devices, from smartphones to ovens to cars, as well as in larger equipment used in factories, military bases, energy-production facilities, and more. According to Nichols, these semiconductors usually have flaws at the atomic level, due either to manufacturing mistakes or to inherent defects in raw materials. Many defects start out as benign, but they can become activated when they absorb energy, such as from electrical currents. Once activated, the defects can cause electrons to scatter, reducing the semiconductor’s electrical output and degrading the performance of the device. Semiconductors can have multiple types of defects, and each defect can have a unique probability that it will activate or cause the device’s performance to degrade.
In the past, this probability (called the scattering cross section) had to be determined through real-world experimentation, which involves simplified equations. Nichols’s work builds on Vanderbilt-developed computer code that provided a way to predict the scattering cross section of a defect using theoretical physics, which allows for much more precise predictions. Vanderbilt researchers have shown a proof of concept for the code, by using it to find the scattering cross section of a simple defect in silicon, a common semiconductor material. Nichols hopes to expand the code to make it more flexible, so that it can be used to predict the scattering cross section for a wider variety of materials and defect types. She said the DOE zeroed in on her research because she is developing original code rather than only using existing code. Her work demonstrates a dual expertise in both computer science and a core scientific field—a skill set the DOE increasingly needs, as today’s scientific problems become too complex to solve on whiteboards.
Because of the duality of her research, Nichols also said she has benefited tremendously from the collaborative environment in the College of Arts and Science. She has two advisers: Kalman Varga, a computational physicist, and Sokrates Pantelides, a theoretical physicist. Varga helps her with computational dilemmas and the “nitty-gritty” of building a functional codebase, while Pantelides highlights opportunities for collaborating with experimentalists and engineers.
“That’s a perspective I’ve never seen. Before this, I’ve just met physicists who were zoomed into a specific project, and nobody really cared about the actual application of it. It feels like I’m doing meaningful work rather than just academic exercises,” Nichols said.
That broader-based perspective has helped Nichols appreciate the true scope of physics. Her coursework at Vanderbilt has involved subjects, such as device physics, that she hadn’t previously studied and didn’t expect to enjoy. The classes, however, showed her how such subjects intersect with her own work and add new dimensions to her research.
“With physics, you don’t have to come to the project knowing everything you need to know,” she said. “The great thing about computational work is that it pulls from so many different pieces. You learn everything along the way, and you don’t have to be an expert to start with. You can investigate anything using physics.”